Radio Telescope: Definition, How it Works, Use, Comparison
Radio telescopes are specialized astronomical instruments used to detect and study radio waves emitted by objects in space. These instruments consist of large antennas or curved dishes that collect and focus radio waves towards receivers. Radio telescopes operate by capturing electromagnetic waves across a wide range of wavelengths, from millimeters to meters. Parabolic dishes reflect collected radio waves onto a focal point, where feed horns focus signals onto receivers for amplification.
Astronomers use radio telescopes to observe and analyze planets, comets, gas clouds, stars, and galaxies. Radio telescopes measure surface temperatures of astronomical objects by analyzing emitted radio waves. The Sun’s surface temperature of approximately 5500°C (10,000°F) has been determined using radio telescope observations. Radio telescopes gather crucial astronomical data for scientific research, including distances to celestial objects and object speeds.
Radio telescopes differ from optical telescopes in several ways. Radio telescopes detect radio waves with wavelengths between 1-30 cm, while optical telescopes detect visible light with wavelengths between 400-700 nm. Radio telescopes are much larger, operate at -200 degrees Celsius, and study cosmic microwave background radiation. Radio telescopes are more sensitive and less affected by atmospheric conditions than optical telescopes.
The Five-hundred-meter Aperture Spherical Telescope (FAST) is the world’s largest radio telescope. FAST is located in Guizhou, China and was completed in 2016. FAST has a 500-meter diameter and 196,000 square meter collecting area. Other major radio telescopes include the Green Bank Telescope in the USA (100 meters in diameter), the Lovell Telescope in the UK (76 meters in diameter), and the Parkes Radio Telescope in Australia (64 meters in diameter).
Electromagnetic Interference (EMI) is unwanted electromagnetic radiation that disrupts electronic devices, including radio telescopes. EMI interferes with radio telescopes by adding noise to signals and decreasing data resolution. Various human activities and devices generate EMI, including radio transmissions, electrical power lines, and electronic equipment. EMI impacts the quality of observations and data collected by radio telescopes, making analysis and interpretation of astronomical phenomena more challenging for scientists.
What is a radio telescope?
Radio telescope is a specialized astronomical instrument used to detect and study radio waves emitted by objects in space. Radio telescopes consist of a large antenna, a curved dish, that collects and focuses radio waves towards a receiver. Receivers amplify and convert the radio signals into data for astronomers to analyze. Radio telescopes observe distant celestial objects like planets, stars, galaxies, gas clouds, and dust clouds that emit radio frequencies rather than visible light. Radio telescopes operate day and night, providing high sensitivity to faint signals and high resolution to observe fine details across a wide range of radio and microwave frequencies.
Radio telescope antennas are positioned at the focal point of the dish to convert collected radio waves into electrical signals. These signals are then processed by radio telescope receivers, which amplify and analyze the weak radio emissions from distant cosmic sources. Radio telescope receivers are highly sensitive, capable of detecting signals as faint as a few Janskys.
Radio telescope arrays combine multiple antennas to function as a single, more powerful instrument. Arrays improve resolution and sensitivity, allowing astronomers to observe celestial objects with greater detail. The Very Large Array (VLA) in New Mexico consists of 27 individual antennas working together to achieve high-resolution imaging.
Radio telescope observations enable the study of objects invisible to optical telescopes. Astronomers use radio telescopes to detect emissions from stars, galaxies, pulsars, quasars, and other cosmic phenomena. Radio telescope astronomy has contributed significantly to our understanding of the universe, revealing information about objects obscured by dust and gas.
Radio telescope signal processing involves analyzing collected data to extract meaningful information about celestial objects. This process helps identify and characterize cosmic sources, determining their temperature, composition, and motion. Radio telescope images produced from this data reveal the structure and behavior of astronomical objects, providing insights into galaxy formation, star evolution, and the nature of black holes.
Radio telescopes have made numerous groundbreaking discoveries. They detected the cosmic microwave background radiation, providing evidence for the Big Bang theory. Radio telescopes discovered pulsars, fast-rotating neutron stars that emit regular pulses of radio waves. These instruments play a crucial role in the search for extraterrestrial intelligence (SETI) by scanning the sky for potential artificial signals.
How do radio telescopes work?
Radio telescopes observe the sky by collecting radio waves emitted by celestial objects. Parabolic dishes reflect collected radio waves onto a focal point. Feed horns focus signals onto receivers for amplification. Receivers amplify weak radio signals to detectable levels. Electronics convert amplified signals into digital format for processing. Computers process digital signals using sophisticated algorithms.
Large dishes concentrate radio waves to detect fainter signals. Multiple antennas receive radio signals from different parts of the sky. Radio telescopes capture electromagnetic waves across a wide range of wavelengths. Radio telescopes pick up signals billions of times weaker than other telescopes. Radio telescopes receive waves that carry information about celestial objects’ properties.
Radio interferometers combine multiple telescopes to form larger virtual telescopes. Combined telescopes improve resolution of radio observations. Radio telescopes work together in arrays to achieve higher sensitivity. Radio telescopes have capabilities to detect extremely weak signals. Radio telescopes help researchers observe objects invisible to optical telescopes.
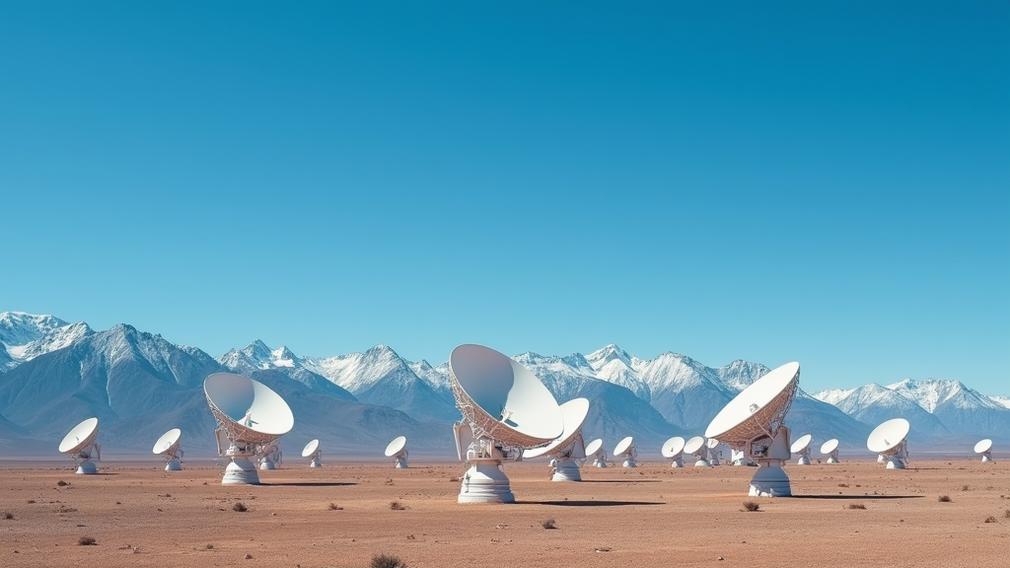
Why can radio telescopes be used 24 hours a day?
Radio telescopes operate 24 hours a day without sunlight interference. Radio waves penetrate clouds and Earth’s atmosphere, enabling continuous observation. Sky sources are observed regardless of time or weather conditions. Radio telescopes function in overcast skies and various atmospheric conditions. Automated systems and advanced technologies support round-the-clock operation.
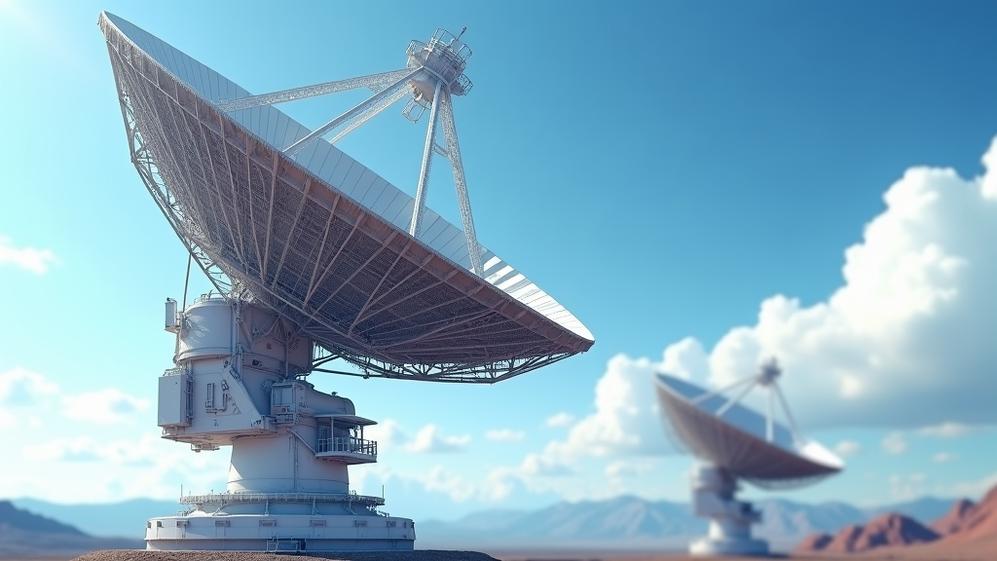
Radio telescopes have continuous operation capabilities. Visible light is not required for radio astronomy, enabling round-the-clock observations. Radio telescopes operate day and night without interruption. The Sun is a weak source of radio waves, allowing unimpeded observations of distant celestial objects. 24-hour monitoring is possible without breaks, providing comprehensive data collection. Observations remain uninterrupted throughout the day-night cycle, allowing for continuous tracking of celestial events.
What astronomical objects can radio waves detect?
The astronomical objects that radio waves can detect are listed below.
- Planets like Jupiter and Saturn (10-100 MHz)
- Comets (1-10 GHz)
- Giant clouds of gas and dust (1-100 GHz)
- Stars, including red giants and supergiants (1-100 GHz)
- Galaxies (100 MHz-10 GHz)
- Pulsars (100 MHz-10 GHz)
- Supernova remnants (100 MHz-10 GHz)
- Active galactic nuclei (100 MHz-100 GHz)
- Black holes (100 MHz-100 GHz)
- Quasars (100 MHz-100 GHz)
Radio waves detect stars, galaxies, planets, comets, pulsars, supernova remnants, gas clouds, dust clouds, active galactic nuclei, cosmic microwave background radiation, and giant molecular clouds. Astronomers use radio telescopes to study object properties like temperature, composition, and motion. Radio astronomy revolutionized understanding of the universe by allowing study of objects invisible to optical telescopes.
Stars, including red giants and supergiants, emit radio waves in the range of 1-100 GHz due to stellar winds and coronal activity. Galaxies emit radio waves at frequencies around 100 MHz-10 GHz through synchrotron radiation from relativistic electrons. Pulsars emit intense beams of radio waves in the range of 100 MHz-10 GHz. Supernova remnants produce radio waves at frequencies around 100 MHz-10 GHz through synchrotron radiation as shock waves accelerate particles.
Active galactic nuclei emit radio waves at frequencies around 100 MHz-100 GHz through various mechanisms, including synchrotron radiation and free-free emission. Black holes influence their surroundings to emit radio waves at frequencies around 100 MHz-100 GHz through accretion processes and relativistic jets. Quasars emit radio waves at frequencies around 100 MHz-100 GHz primarily through synchrotron radiation. Radio waves have wavelengths longer than 1 mm and frequencies lower than 300 GHz, providing valuable information about the physical properties, behavior, and evolution of these astronomical sources.
What are radio telescopes used for?
Radio telescopes are powerful instruments used to study radio waves emitted by astronomical objects in space. Astronomers employ radio telescopes to observe and analyze planets, comets, gas clouds, stars, and galaxies. Radio telescopes detect and record radio waves from distant celestial bodies, providing valuable data about their properties and behavior.
Radio telescopes measure surface temperatures of astronomical objects by analyzing emitted radio waves. The Sun’s surface temperature of approximately 5500°C (10,000°F) has been determined using radio telescope observations. Radio telescopes observe objects obscured by cosmic dust and gas clouds, allowing scientists to study regions invisible to optical telescopes. Jupiter’s intense radio emissions from its magnetic field have been studied extensively using radio telescopes.
Radio telescopes gather crucial astronomical data for scientific research. Distances to celestial objects are determined by measuring radio wave travel time or using the “redshift” method. Object speeds are measured by observing the Doppler shift of radio waves emitted by moving celestial bodies. Radio telescopes analyze radio wave emissions to extract information about the composition, structure, and evolution of astronomical objects.
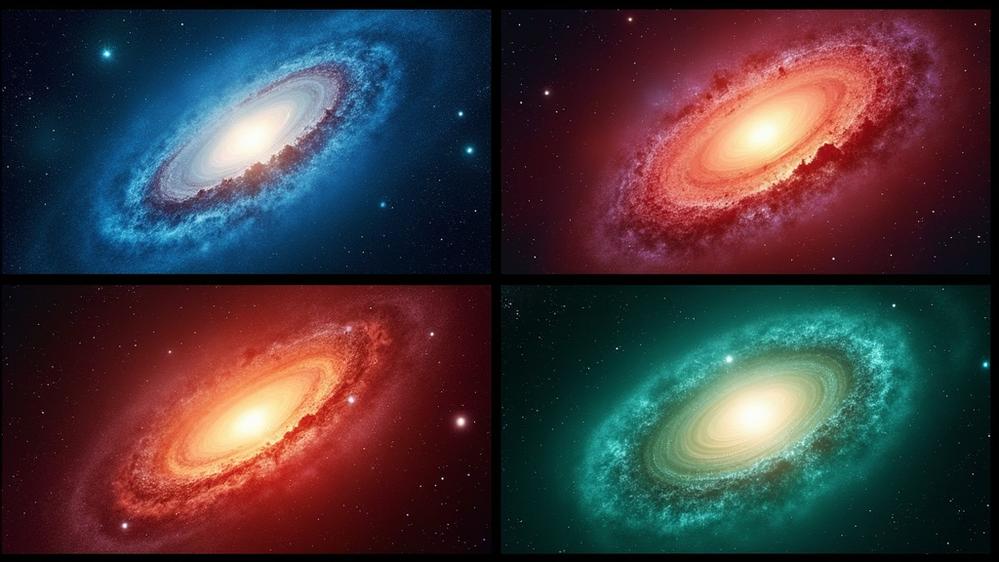
How do radio telescopes help advance our knowledge of our universe?
Radio telescopes detect invisible cosmic objects using radio waves. Astronomers study distant stars, galaxies, black holes, and planets through radio light. Radio astronomy enhances understanding of the universe’s origin and evolution. Advanced telescopes like ALMA in Chile observe star and planet formation in unprecedented detail. Radio telescopes revolutionize cosmic knowledge by examining composition, structure, and motion of celestial bodies.
Radio telescopes study the properties of celestial objects by examining their radio emissions. Scientists determine the composition, structure, and motion of cosmic objects through radio wave analysis. Magnetic fields of celestial bodies are explored using radio telescope data. Continuum radiation and spectroscopic features are measured to gain insights into object characteristics.
Radio telescopes enable the study of hydrogen atoms in the universe. Astronomers map galactic gas distribution by observing hydrogen radio emissions. Star formation processes are investigated through hydrogen observations with radio telescopes.
Radio telescopes explore invisible phenomena like black holes and dark matter. Objects obscured by gas and dust are observed, providing a unique view of the universe not visible to optical telescopes. Radio telescopes have detected gravitational waves and discovered exoplanets.
Radio telescopes have revolutionized astronomy and expanded our understanding of the universe. Cosmic microwave background radiation was discovered using radio telescopes. New celestial objects like pulsars and quasars were first identified through radio astronomy. Radio telescopes measure cosmic distances precisely and map the structure of the Milky Way galaxy and the universe.
How do radio telescopes compare to optical telescopes?
Radio telescopes detect radio waves with wavelengths λ between 1-30 cm. Optical telescopes detect visible light with wavelengths λ between 400-700 nm. Radio telescopes are much larger, operate at -200 degrees Celsius, and study cosmic microwave background radiation. Optical telescopes observe stars and planets. Radio telescopes are more sensitive and less affected by atmospheric conditions.
Wavelength and energy levels distinguish these two types of telescopes. Radio telescopes detect radio waves with wavelengths ranging from millimeters to meters. Optical telescopes capture visible light with wavelengths between 380-700 nanometers.
Detection and imaging methods vary between radio and optical telescopes. Radio telescopes produce false-color maps representing radio wave intensity. Optical telescopes generate visible pictures of celestial objects as seen by the human eye.
Observation capabilities differ based on the type of telescope. Radio telescopes operate continuously, functioning during both day and night without interference. Optical telescopes perform optimally during clear daytime conditions, limited by atmospheric disturbances and light pollution.
Focusing methods are distinct for each telescope type. Radio telescopes utilize large dish antennas to concentrate radio waves onto receivers. Optical telescopes employ lenses or mirrors to focus visible light onto detectors or eyepieces.
Wavelength range and temperature sensitivity vary between the two telescope types. Radio telescopes observe one wavelength at a time using narrowband receivers, sensitive to cooler objects like interstellar gas. Optical telescopes view multiple wavelengths simultaneously with broadband detectors, demonstrating sensitivity to hotter objects such as stars.
What is the largest radio telescope in the world?
The Five-hundred-meter Aperture Spherical Telescope (FAST) is the world’s largest radio telescope. FAST is located in Guizhou, China. Chinese scientists completed FAST in 2016. FAST sits in a natural depression in the mountains. FAST has a 500-meter diameter and 196,000 square meter collecting area. People call FAST the Tianyan or “Heavenly Eye”.
Major radio telescopes vary in size, with FAST leading the pack. The Arecibo Observatory in Puerto Rico held the second position with a diameter of 305 meters until its collapse in 2020. The Green Bank Telescope in the USA measures 100 meters in diameter. The Lovell Telescope in the UK has a diameter of 76 meters. The Parkes Radio Telescope in Australia spans 64 meters in diameter.
A radio telescope is a specialized antenna designed to detect radio waves emitted by celestial objects. Radio telescopes consist of large dish-shaped antennas that collect and focus radio waves from space. Scientists analyze these radio waves to gain insights into the composition, structure, and behavior of celestial objects. Radio telescopes enable the study of the universe in ways impossible with optical telescopes alone.
What is emi and why does emi interfere with radio telescopes?
Electromagnetic Interference (EMI) is unwanted electromagnetic radiation disrupting electronic devices. EMI interferes with radio telescopes by adding noise to signals and decreasing data resolution. Radio telescopes use portions of the electromagnetic spectrum utilized by other devices. Electrical equipment, telecommunication systems, and natural radiations cause EMI. Radio telescopes are located remotely to mitigate EMI effects.
EMI disrupts signals and causes malfunction in telescope electronics. Various human activities and devices generate EMI, including radio transmissions, electrical power lines, and electronic equipment. These sources produce electromagnetic radiation that spills frequencies into the radio frequency range used by telescopes. The spilled frequencies produce disturbances that interfere with radio telescopes’ observations.
EMI generates radio frequency interference (RFI) that overpowers weak radio signals from celestial objects. RFI makes signal detection difficult by decreasing the signal-to-noise ratio of observations. EMI impacts the quality of observations and data collected by radio telescopes. The reduced data quality makes analysis and interpretation of astronomical phenomena more challenging for scientists.
Radio astronomers employ several techniques to mitigate EMI effects. Radio telescopes use shielding to prevent EMI entry and filter radio signals to remove interference. Telescopes operate at specific frequencies that are less prone to EMI. Despite these measures, EMI remains a significant challenge in radio astronomy, affecting the accuracy and reliability of scientific observations.