Quasar: Definition, Clocks, Difference, Facts, Discovery
Quasars are exceptionally luminous active galactic nuclei powered by supermassive black holes at galaxy cores. They emit massive amounts of electromagnetic radiation across the spectrum, outshining entire galaxies. Quasar light is visible from billions of light-years away, offering insights into early universe formation and black hole evolution. Most observed quasars have redshifts greater than 1, with the most distant quasar having a redshift of 7.54.
Quasars act as cosmic clocks for researchers tracking time across the universe. Their consistent brightness patterns vary like the ticking of a clock, allowing measurement of vast cosmic distances. Quasar observations reveal the universe’s expansion rate at various epochs, ranging from 50-100 km/s/Mpc in early times to 70-80 km/s/Mpc later. Quasar cosmic clocks demonstrate that time passed 5 times slower in the early universe, confirming predictions from Einstein’s theory of general relativity.
Quasars are rapidly growing supermassive black holes at the cores of hyperactive galaxies, while black holes are regions of extreme gravitational pull. Quasars release enormous amounts of energy through accretion, efficiently converting mass to energy. Black holes store energy in their mass and do not release energy on their own. Quasars are visible from vast cosmic distances up to 13 billion light-years away, while black holes are not directly observable.
Quasars are the brightest objects in the universe, emitting energy up to a trillion times brighter than our Sun. They produce radiation across the entire electromagnetic spectrum, including visible light, ultraviolet, X-rays, and gamma rays. Quasars surround supermassive black holes at the centers of galaxies, forming accretion disks as material falls toward the black hole. The closest quasar is located about 1.3 billion light-years away.
Quasars were first discovered in the early 1960s. Alan Sandage discovered 3C 48 in 1960, and Maarten Schmidt discovered the first confirmed quasar, 3C 273, in 1962. Over 200,000 quasars are known to astronomers today, each providing valuable insights into the early universe and galaxy formation. Quasars were most active during the epoch of reionization 13 billion years ago.
Quasars emit blue-white light with wavelengths between 400-500 nanometers. Distant quasars appear redder due to cosmic expansion and intervening dust. Quasar light is bluer and more intense than common lighting options, resembling high-energy plasma. Quasar brightness can outshine entire galaxies of stars, with some quasars shining hundreds of times brighter than typical stars.
What is a quasar in astronomy?
Quasars are exceptionally luminous active galactic nuclei. Supermassive black holes power quasars at galaxy cores. Active galactic nuclei emit massive amounts of electromagnetic radiation across the spectrum. Quasars outshine entire galaxies. Quasars release energy visible from billions of light-years away. Quasars offer insights into early universe formation and black hole evolution.
Quasar light includes radio waves, infrared, visible, ultraviolet, X-rays, and gamma rays. Polarized light emitted by quasars indicates passage through intense magnetic fields. Quasar jets are powerful streams of energetic particles emitted from the vicinity of the supermassive black hole. These jets can extend thousands of light-years in length. Quasars reside in the distant universe, with most observed quasars having redshifts greater than 1. The most distant quasar observed has a redshift of 7.54, seen as it existed 700 million years after the Big Bang.
Quasar redshifts indicate rapid movement away from Earth and reveal properties of the early universe. Astronomers use quasars as “cosmic lighthouses” to examine the universe at high redshifts. Quasar host galaxies can be spiral, elliptical, or irregular. The quasar nucleus is the region surrounding the supermassive black hole, shrouded by dust and gas. Quasar science draws on astronomy, astrophysics, and cosmology to study universe properties at high redshifts.
Maarten Schmidt discovered the first quasar in 1959. Thousands of quasars have been discovered since then. Quasars formed in the early universe through the growth of supermassive black holes. Astronomers label quasars as “QSO” (Quasi-Stellar Object) or “AGN” (Active Galactic Nucleus). Quasar parts include the supermassive black hole, accretion disk, and energetic particle jets. Quasars undergo several evolutionary stages, including black hole formation, accretion disk growth, and jet emission.
What are quasar cosmic clocks?
Quasars act as cosmic clocks for researchers tracking time across the universe. Supermassive black holes power these luminous objects at galaxy centers. Quasars vary in brightness over time, allowing measurement of vast cosmic distances. Some quasars outshine the entire Milky Way, visible from over 100 billion light-years away. Researchers study quasars to understand universe evolution.
Quasars exhibit consistent brightness patterns that vary like the ticking of a clock. The relationship between quasar luminosity and size allows astronomers to estimate distances accurately. Time dilation effects due to cosmic expansion cause quasar light to appear slowed down, providing insights into the universe’s age and evolution.
Astronomers measure cosmic distances by analyzing quasar light curves and spectral lines at different redshifts. Quasar observations reveal the universe’s expansion rate at various epochs, ranging from 50-100 km/s/Mpc in early times to 70-80 km/s/Mpc later. Quasar cosmic clocks enable testing of gravity theories and cosmological models by providing data on universe conditions during the first 5% of its current age.
Quasars formed approximately 700 million years after the Big Bang, allowing study of the early universe. Observations of quasars at 13 billion year look-back times show the universe’s state when it was only 1 billion years old. Quasar cosmic clocks demonstrate that time passed 5 times slower in the early universe, confirming predictions from Einstein’s theory of general relativity.
How are quasar cosmic clocks used to study expansion of universe?
Quasars serve as cosmic clocks for studying universe expansion. Scientists measure quasar light travel time over billions of years. Observations at different times confirm accelerating expansion. Einstein’s theory of relativity predicts time dilation for moving quasars. Redshift analysis of quasar spectra demonstrates expanding space. Quasar measurements validate Einstein’s predictions and reveal the universe’s 13.8-billion-year age.
Quasars help confirm relativity theories on a cosmic scale. Shapiro (1964) used quasars as cosmic lighthouses to validate universe models. Hubble (1929) observed cosmological redshift in quasars, revealing their distance and velocity. Quasars serve as cosmic mileposts for expansion studies, providing data across immense timescales.
Scientists use quasars to unlock secrets of the early universe. Blandford (2019) studied quasars to reveal the formation of supermassive black holes and growth of galaxy clusters. Liske (2008) measured the passage of time in distant regions using quasars. The Planck Collaboration (2020) calculated the universe’s age as 13.8 billion years based on quasar data.
Astronomers rewind the cosmic clock to understand the universe’s expansion history. Riess (1998) studied quasar properties at different redshifts to determine expansion rates at various epochs. Hawkins (2010) used observed slowing of some quasars to study dark energy. Quasars enable scientists to track universal expansion over billions of years, providing crucial insights into cosmic evolution.
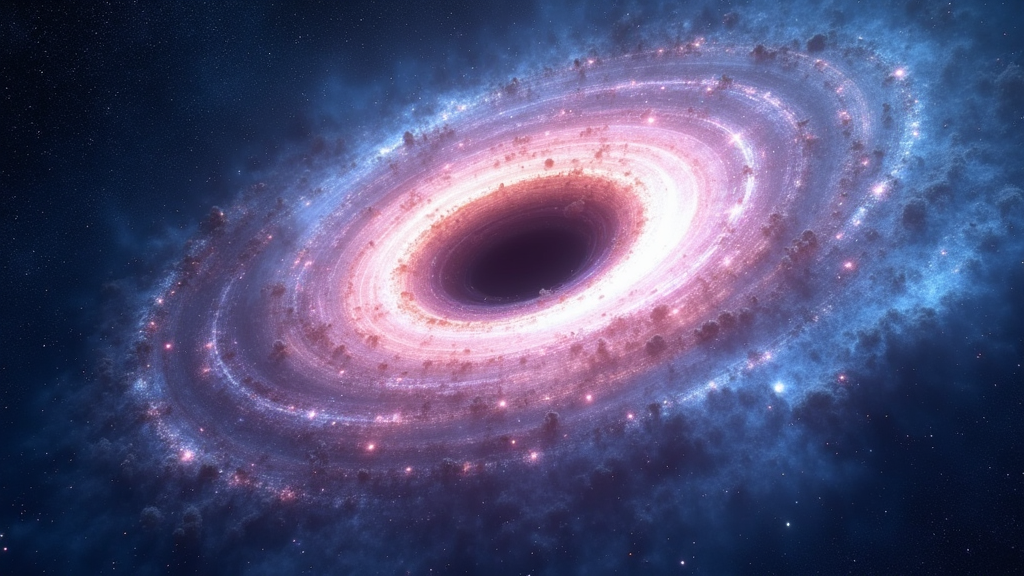
What is the difference between a quasar and a black hole?
Quasars are rapidly growing supermassive black holes at the cores of hyperactive galaxies. Black holes are regions of extreme gravitational pull. Supermassive black holes power quasars by accreting material. Accretion disks form dense rings of ultrahot gas around black holes. Quasars outshine entire galaxies. Not all black holes are quasars.
Quasars are powered by supermassive black holes at galactic centers. Supermassive black holes have masses millions or billions of times the Sun’s mass. Quasars include the central black hole and its surrounding bright accretion disk. Black holes are compact objects without visible surrounding material, encircled only by an ergosphere.
Quasars release enormous amounts of energy through accretion, efficiently converting mass to energy. Black holes store energy in their mass and do not release energy on their own. Quasars are visible from vast cosmic distances up to 13 billion light-years away. Black holes are not directly observable and scientists infer their presence indirectly.
Quasars grew rapidly in the early universe and represent an early stage of galactic evolution. Black holes have formed throughout cosmic history and grow through accretion over long periods. Quasars show us glimpses of the early universe, existing as far back as 13 billion years ago. Black holes exist at various ages and growth stages in the present-day universe.
Astronomers observe quasars directly as among the brightest objects in the universe. Scientists detect black holes indirectly through their effects on nearby objects. Quasars are distinct from pulsars and gamma-ray bursts, with blazars forming a subclass of quasars with relativistic jets.
What is the difference between a quasar and a gamma ray burst?
Quasars emit energy across the electromagnetic spectrum for millions of years. Gamma ray bursts release enormous energy as gamma rays in milliseconds to minutes. Quasars are powered by supermassive black holes in galactic centers. Gamma ray bursts result from massive star explosions or compact object mergers. Quasars appear star-like with steady brightness. Gamma ray bursts are more energetic, explosive events.
Quasars occur more in the observable universe, with hundreds of thousands known. Gamma-ray bursts are rarer, with only hundreds detected yearly. Quasars appear as reddened point sources across multiple wavelengths from Earth. Gamma-ray bursts manifest as brief gamma-ray flashes followed by afterglows in other wavelengths.
Quasars exhibit variability over days to years due to changes in accretion rates. Gamma-ray bursts show rapid variability within milliseconds to seconds caused by intrinsic processes. Supermassive black holes in galactic centers power quasars through accretion of surrounding material. Collapsing massive stars or merging compact objects cause gamma-ray bursts through catastrophic events.
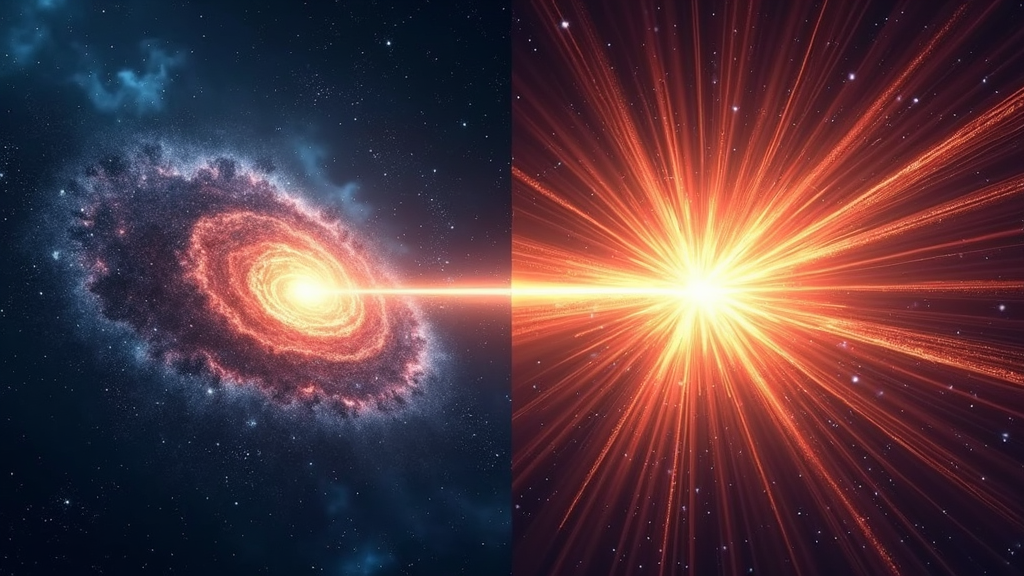
What is the difference between a quasar and a blazar?
Quasars and blazars differ in jet orientation relative to Earth. Quasars’ jets are observed at larger angles, revealing broad emission lines from accretion disks. Blazars’ jets point almost directly at us, appearing brighter and more variable. Both harbor supermassive black holes in active galactic nuclei. Viewing angle creates apparent differences in these high-energy objects.
Luminosity and variability distinguish quasars from blazars. Quasars emit up to 10^47 erg/s and show luminosity changes over days to weeks. Blazars emit up to 10^48 erg/s and exhibit rapid variability, with luminosity changes on timescales of minutes to hours. Blazars appear extremely bright due to relativistic beaming effects.
Energy output and radiation type differ between quasars and blazars. Quasars emit radiation across radio, optical, UV, X-ray, and gamma-ray wavelengths. Blazars dominate in the gamma-ray regime, with some emitting more than 10^48 erg/s in this range. Blazars have higher jet density than quasars, leading to more efficient particle acceleration and increased radiation production.
Power, density, and frequency of bursts vary between quasars and blazars. Quasars have more stable energy output and show moderate variability of up to 20% over weeks to months. Blazars exhibit frequent bursts of activity with high-energy flares, showing flux changes of up to 50% over hours to days. Blazars have multiple flares per year, while quasars do not exhibit the same level of bursting activity.
Directness towards Earth impacts observed properties of quasars and blazars. Quasars have larger viewing angles and less direct streams towards Earth. Blazars have a more direct angle of stream towards Earth, resulting in higher observed luminosity and variability. Relativistic beaming enhances radiation in the observer’s direction for blazars.
Classification within AGN categorizes quasars and blazars differently. Quasars represent a broader class of AGN observed at various angles. Blazars are a subset of AGN where we look straight into the jet. Quasars are classified as radio-quiet or radio-loud. Blazars are divided into subclasses like BL Lac objects and flat-spectrum radio quasars.
Quasars were the first type of AGN to be discovered. The first quasar (3C 273) was detected in 1959. Blazars were not well-defined as a class until the 1990s. The launch of the Compton Gamma-Ray Observatory led to the detection of gamma-ray loud blazars.
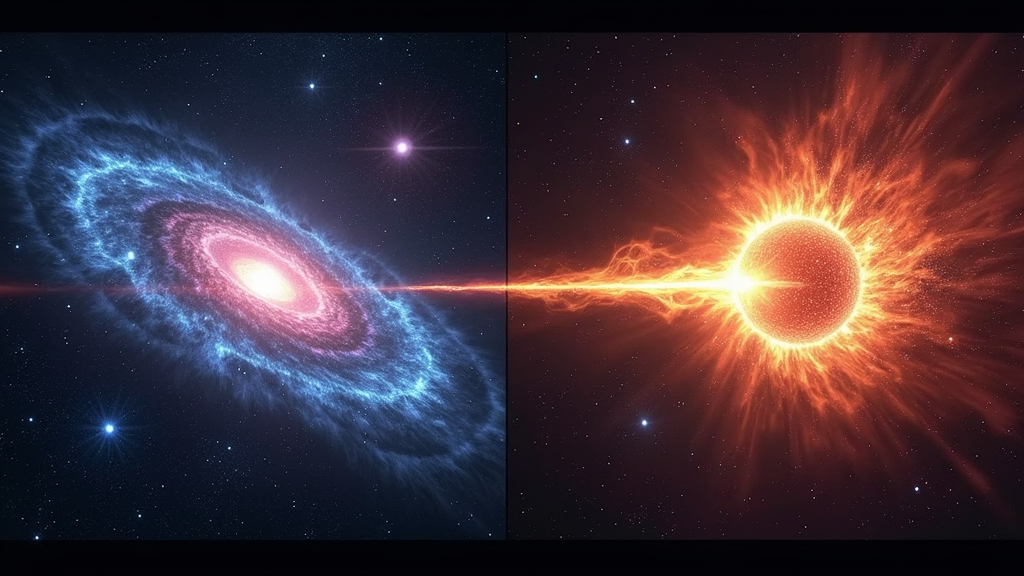
What is the difference between a quasar and a pulsar?
Quasars are extremely bright, distant active galactic nuclei powered by supermassive black holes. Quasars outshine entire galaxies. Pulsars are rotating, highly magnetized neutron stars. Pulsars emit precise electromagnetic pulses. Quasars measure billions of kilometers in diameter. Pulsars measure 10-20 kilometers. Quasars exhibit variable brightness. Pulsars maintain stable pulse periods.
Quasars reside billions of light-years away from Earth. Pulsars exist within the Milky Way or nearby galaxies. Quasars originate as active galactic nuclei. Pulsars originate as remnants of dead stars. Quasars emit massive amounts of energy across the entire electromagnetic spectrum. Pulsars emit beams of radiation, primarily in radio waves, X-rays, and visible light.
Pulsars spin rapidly, rotating hundreds of times per second. Quasars do not necessarily rotate. Pulsars possess strong magnetic fields, trillions of times stronger than Earth’s. Quasars lack defined magnetization. Pulsars have a layered structure with a crust, mantle, and core. Quasars lack a defined internal structure.
Pulsars are classified as stars. Quasars are classified as active galactic nuclei. Quasars power galactic cores through matter accretion. Pulsars form from supernova explosions. Quasars have masses millions or billions of times that of the sun. Pulsars have incredibly dense compositions.
What are fun facts about quasars?
Fun facts about quasars are listed below.
- Quasars are the brightest objects in the universe, outshining entire galaxies.
- They emit energy up to a trillion times brighter than our Sun.
- Quasars produce radiation across the entire electromagnetic spectrum, including visible light, ultraviolet, X-rays, and gamma rays.
- Quasars surround supermassive black holes at the centers of galaxies.
- Quasars form accretion disks as material falls toward the black hole, generating intense heat that fuels their incredible luminosity.
- Quasars feed messily on gas and dust from their surroundings, contributing to their extreme brightness and energy output.
- Quasars are among the most distant objects observable in the universe.
- The closest quasar to Earth is located about 1.3 billion light-years away.
- Quasars exhibit broadened spectral lines due to the intense gravitational field of the black hole.
- Quasars power entire galaxies by regulating their growth and preventing new star formation.
- Quasars play a key role in galaxy formation and evolution.
Quasars give off enormous energy, outshining normal galaxies by a trillion times. Distant objects in the universe, quasars are brighter than 100 billion suns. Quasars give energy across the entire electromagnetic spectrum. Supermassive black holes power these incredibly luminous objects. The closest quasar is 1.3 billion light-years from Earth.
Quasars surround supermassive black holes at the centers of galaxies. They form accretion disks as material falls toward the black hole, generating intense heat that fuels their incredible luminosity. Quasars feed messily on gas and dust from their surroundings, contributing to their extreme brightness and energy output.
Quasars are among the most distant objects observable in the universe, with the closest quasar located about 1.3 billion light-years away. They exhibit broadened spectral lines due to the intense gravitational field of the black hole. Quasars power entire galaxies by regulating their growth and preventing new star formation, playing a key role in galaxy formation and evolution.
When were quasars discovered?
Quasars were first discovered in the early 1960s. Astronomers initially identified quasars as small, bright radio sources in the 1950s. Alan Sandage discovered 3C 48 in 1960. Maarten Schmidt discovered the first confirmed quasar, 3C 273, in 1962. These discoveries revolutionized astronomy by revealing extremely luminous, distant active galactic nuclei.
Astronomers have discovered thousands of quasars since the 1960s, expanding our understanding of the universe. Over 200,000 quasars are known to astronomers today, each providing valuable insights into the early universe and galaxy formation. Quasars were most active during the epoch of reionization 13 billion years ago, playing a key role in early galaxy formation and evolution. Some quasars are over 13 billion light-years away from Earth, serving as cosmic lighthouses for studying the distant universe.
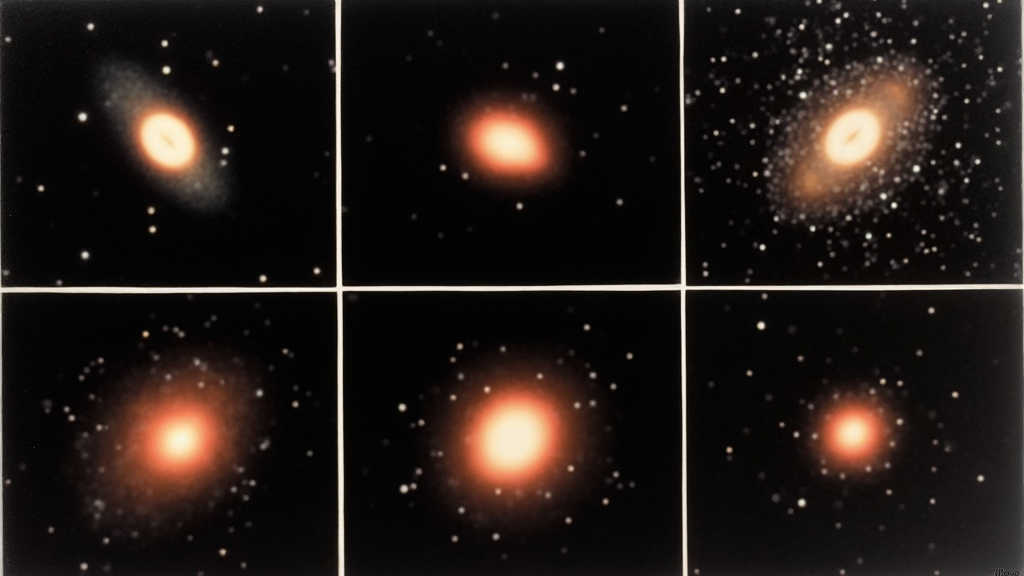
How were quasars discovered?
Quasars were discovered in the 1950s through radio surveys. Astronomers identified small, bright objects emitting large amounts of radiation. Radio telescopes like Parkes enabled detection of early quasars such as 3C 273 in August 1962. Quasars appeared as faint objects through telescopes, smaller and brighter than galaxies. Lovell observed quasar objects using radio telescopes.
Alan Sandage photographed a distant star system in 1960, later identified as a quasar. Maarten Schmidt published a groundbreaking revelation about quasar 3C 273 in 1963. Schmidt identified 3C 273 as a quasi-stellar radio source at an immense distance from Earth. Radio astronomy began advancing rapidly in the 1960s, leading to the location of more radio sources classified as quasars.
Scientists recognized some radio galaxies as quasars during this period. Researchers studied quasar 3C 48 extensively, identifying powerful radio emission from these objects. Observers noted star-shaped objects in the optical spectrum associated with these radio sources. Quasars occur in the distant universe, powered by supermassive black holes at their centers.
How are quasars formed?
Quasars form when supermassive black holes at galaxy centers accrete massive amounts of gas. Galaxy mergers trigger gas infall towards central black holes. Accreting gas forms hot disks around black holes, emitting huge amounts of light. Edwin Salpeter and Yakov Zeldovich proposed this model in the 1960s. Observational evidence has since confirmed their theories.
Galactic interactions play a crucial role in quasar formation. Galaxy mergers trigger the accumulation of gas at the centers of galaxies, as demonstrated by Hopkins et al. (2006) in their simulations. Violent collisions between galaxies compress and shock gas, leading to intense star formation and rapid black hole growth, according to Mihos & Hernquist (1996). Cold gas streams, rich in molecular gas, provide a steady fuel supply for growing black holes, as shown by Dekel et al. (2009) in their research.
The accretion process is central to quasar formation. Gas piles up around the supermassive black hole, forming a dense accretion disc as described by Shakura & Sunyaev (1973) in their influential model. Material from the accretion disc falls into the black hole, releasing enormous amounts of gravitational energy. This energy release creates the intense radiation output characteristic of quasars, as explained by Rees (1984) in his study of quasar energetics.
Quasars formed early in the universe’s history. Fan et al. (2006) discovered quasars existing just 700 million years after the Big Bang. These early quasars played a significant role in shaping galaxy evolution. Quasars are known to be among the brightest objects in the universe, outshining entire galaxies of stars. The first quasar was reported by Schmidt in 1963, leading to the discovery of thousands more in subsequent years.
What color is a quasar’s light?
Quasars emit blue-white light with wavelengths between 400-500 nanometers. Distant quasars appear redder due to cosmic expansion and intervening dust. Interstellar reddening occurs as light travels through space. Richards et al. (2003) and Hopkins et al. (2007) studied quasar properties, including color variations related to redshift, luminosity, and dust presence.
Quasar light exhibits specific color temperatures comparable to artificial lighting options. 2000K warm white light resembles traditional incandescent bulbs and candlelight. 3000K fixed white light is similar to T8 lamps commonly used in office lighting. 6000K cool white light is produced by fluorescent and LED bulbs, creating bright atmospheres.
Quasars have a blue-white color due to their high-energy emission. Quasar light is bluer and more intense than common lighting options, resembling high-energy plasma. Quasars shine with light hotter than ordinary stars, appearing as brilliant, shining points in the sky. Quasar brightness can outshine entire galaxies of stars, with some quasars shining hundreds of times brighter than typical stars.
How hot is a quasar?
Quasars reach core temperatures of 10 trillion degrees Celsius (18 trillion Fahrenheit). Quasar cores are hotter than star surfaces, which have effective temperatures of 5,000 to 50,000 degrees Celsius. Quasars rank among the highest-energy objects in the universe. Quasar gas disks heat to approximately 10 trillion degrees Celsius in some cases.
The highest recorded temperature for a quasar is 10 trillion degrees Celsius, observed in quasar 3C273 located in the Virgo constellation. This temperature equals 18 trillion degrees Fahrenheit, demonstrating the immense energy release by quasars. The maximum theoretically allowed temperature is 1 trillion kelvin, equivalent to 1 trillion degrees Celsius or 1.8 trillion degrees Fahrenheit. Laws of physics and quantum mechanics impose this fundamental temperature limit.
Quasars exhibit extreme temperatures compared to other celestial bodies. The sun’s surface temperature ranges from 5,000 to 10,000 degrees Celsius, or 9,000 to 18,000 degrees Fahrenheit. Quasar temperatures surpass the sun’s surface temperature by several orders of magnitude. Quasars were most active 10-13 billion years ago, during the early stages of universe formation. Supermassive black holes power quasars through material accretion, releasing tremendous energy across the electromagnetic spectrum.
What is the temperature of quasar 3C273?
Scientists combined telescopes on Earth and in space to measure quasar 3C273’s core temperature. 3C273’s temperature exceeds 10 trillion degrees Celsius, significantly hotter than previously thought possible. The extremely hot accretion disk surrounding the supermassive black hole at the quasar’s center reaches approximately 50 million Kelvin, making 3C273 one of the universe’s brightest objects.
Spectral analysis has yielded different temperature estimates for 3C273. Von Montigny et al. (1997) deduced a temperature of around 21,000 K from fits to the quasar’s optical and ultraviolet spectrum. Paltani et al. (1998) estimated a higher temperature of about 26,000 K using similar spectral fitting techniques.
Temperature estimates for 3C273 vary widely due to different assumptions and methods used in calculations. All estimates consistently indicate that 3C273 is an extremely hot object with temperatures far exceeding those found in normal stars. The core brightness temperature of 3C273 is estimated to be around 10^13 K, reinforcing its status as an exceptionally energetic cosmic phenomenon.
What is the primary source of a quasar’s energy?
Supermassive black holes at the centers of active galaxies power quasars. Black holes feed on humungous quantities of gas and dust. Matter falling into the black hole forms an accretion disk. Accretion disks convert gravitational energy into intense radiation. Quasars outshine entire galaxies, emitting hundreds of times more energy than the Milky Way.
Gravitational potential energy is converted to radiation through the process of accretion. An accretion disk forms around the black hole as surrounding interstellar gas funnels towards the galaxy center. Gas feeding into the black hole occurs as material spirals inward through the accretion disk. Energy is emitted as matter falls into the black hole, heating up due to friction and releasing light across the electromagnetic spectrum.
Quasars exhibit extremely high luminosities, outshining entire galaxies. Quasar energy output ranges from radio waves to gamma rays. Quasar luminosities vary significantly over time, changing by factors of 10 or more. Variations in gas feeding rates into the black hole cause these luminosity changes. Quasar light travels through space for billions of years before reaching Earth, providing glimpses into the distant past of the universe.
What is the size of the region where a quasar’s luminosity is generated?
Quasars generate luminosity from an incredibly compact region, ranging from 10^-5 to 10^-2 light years in size. The smallest known quasar has an emitting region of just 10^-6 light years, approximately 1/1,000th the size of our solar system. Rapidly varying quasars exhibit brightness changes over hours or days, with emitting regions around 10^-5 light years across. Average quasars have larger luminosity regions of about 10^-4 light years. Typical quasars produce their intense radiation from an area spanning 10^-3 light years, 1,000 times smaller than the distance to the nearest star. Large quasars, powered by more massive black holes, have emitting regions up to 10^-2 light years in diameter.
Quasars are powered by supermassive black holes at the centers of galaxies. Accretion disks surrounding these black holes generate the intense radiation observed in quasars. The size of the accretion disk directly correlates with the quasar’s brightness and variability. Quasars vary greatly in luminosity, with some changing brightness by up to 50% in just a few days. Researchers measure quasar sizes in light years, with one light year equaling approximately 9.461 x 10^12 kilometers.
What is the brightest quasar?
3C 273 is the brightest known quasar. This distant quasar shines 100 trillion times brighter than the sun. A supermassive black hole powers 3C 273. The black hole has a mass 6.6 million times that of the sun. 3C 273 is located 2.4 billion light-years away in Virgo constellation.
Can the quasar cause a tsunami on Earth?
Quasars cannot cause tsunamis on Earth. Quasars are located billions of light-years away from our planet, making their direct impact on Earth’s oceans impossible.
Quasar energy dissipates significantly over vast cosmic distances. Quasar radiation intensity at Earth’s surface is approximately 10^-12 W/m^2, which is a tiny fraction compared to the Sun’s energy flux of 1366 W/m^2. Quasar radiation consists primarily of electromagnetic waves, including visible light, ultraviolet radiation, and X-rays. Electromagnetic radiation does not have the physical properties necessary to displace water and generate tsunamis on Earth.
Quasar winds reach speeds of 10,000 km/s but remain confined to the quasar’s vicinity. Earth’s magnetic field and atmosphere effectively deflect or absorb any remnants of quasar winds that might reach our planet. Quasar tsunamis, while occurring within the host galaxies of quasars, do not pose any threat to Earth. Typical tsunami waves on Earth release approximately 10^12 joules of energy, far exceeding the energy flux from distant quasars by many orders of magnitude.
What are quasar examples?
ULAS J1342+0928, a z former distant quasar, has the highest known redshift of 7.54. ULAS Z0041-0159 and Pōniuāʻena are z former distant quasars. QSO J1148+5251 is classified as a distant quasar with z=6.42. 3C 273, a former distant quasar, is one of the brightest at 2.4 billion light-years away. These examples showcase quasars’ diversity.
ULAS J1342+0928 is one of the most luminous objects in the universe, with a luminosity of approximately 4 × 10^14 times that of the Sun. Researchers observe ULAS J1342+0928 as it was about 690 million years after the Big Bang. J1007+2115, known as Pōniuāʻena, is one of the brightest objects in the universe, with a luminosity of around 1.5 × 10^14 times that of the Sun. Astronomers observe J1007+2115 (Pōniuāʻena) as it was about 700 million years after the Big Bang.
ULAS J1120+0641 (ULAS J112001.48+064124.3) is another extremely distant object, with a redshift of z = 7.09. Scientists observe ULAS J1120+0641 as it was about 770 million years after the Big Bang. Researchers estimate ULAS J1120+0641’s luminosity to be around 2.5 × 10^13 times that of the Sun. Quasars are incredibly powerful objects that scientists believe are powered by supermassive black holes at the centers of galaxies.