Spherical Aberration: Definition, Why it Occurs
Spherical aberration is an optical phenomenon where light rays passing through a lens or curved mirror fail to converge at a single focal point. Light rays striking different parts of a lens are refracted at varying angles, causing them to focus at multiple points along the optical axis. Spherical aberration results in distorted or blurry images, significantly reducing the quality of the optical system’s output. Aspheric lenses or mirrors, as well as combinations of lenses with different curvatures, are effective methods for correcting spherical aberration in optical designs.
Spherical aberration occurs in lenses due to their spherical surfaces. Incoming light rays strike curved lens surfaces at different angles, causing rays passing through larger angles to refract more than those at smaller angles. Light rays passing through the outer parts of the lens focus closer to the lens surface, while rays passing through the central part focus farther away. The focal length difference between central and edge light rays defines spherical aberration. A 50 mm focal length lens with a 25 mm diameter experiences significant image quality loss with 1 mm of spherical aberration.
Spherical aberration occurs in optical systems with spherical-shaped lenses and elements. Lenses operating at maximum aperture exhibit more pronounced spherical aberration. Oblique rays enter the lens at an angle during light passage and are refracted more than central rays. Large apertures in optical systems increase spherical aberration. Telescopes with spherical surfaces cause light rays to focus at different points, resulting in significant image distortion.
Spherical aberration differs from chromatic aberration in cause and effect. Spherical aberration results from lens shape, while chromatic aberration stems from varying light wavelengths. Spherical aberration manifests as a circular blur or halo around the image, while chromatic aberration appears as color fringing at high-contrast edges. Aperture size primarily affects spherical aberration, while optical material quality mainly influences chromatic aberration. Spherical aberration occurs with both monochromatic and polychromatic light sources, whereas chromatic aberration is significant only with polychromatic light sources.
What is spherical aberration?
Spherical aberration is an optical phenomenon where light rays passing through a lens or curved mirror fail to converge at a single focal point. Spherical aberration occurs commonly in optical systems with spherical surfaces, such as microscopes, telescopes, and camera lenses. Light rays striking different parts of a lens are refracted at varying angles, causing them to focus at multiple points along the optical axis. Spherical aberration results in distorted or blurry images, significantly reducing the quality of the optical system’s output. Aspheric lenses or mirrors, as well as combinations of lenses with different curvatures, are effective methods for correcting spherical aberration in optical designs.
Spherical aberration causes include the inherent shape of spherical lenses and mirrors. The spherical shape leads to varying refraction and reflection across the surface. Spherical aberration occurs in all types of spherical optical elements, including lenses, mirrors, and the cornea of the eye. Larger apertures increase spherical aberration by utilizing more of the lens periphery. Spherical aberration in telescopes significantly impacts image quality, in large-aperture instruments. Telescope manufacturers use aspheric lenses, corrector plates, and aperture masks to mitigate spherical aberration.
Spherical aberrations are more pronounced at larger apertures and longer focal lengths. Positive spherical aberration occurs when the lens or mirror is too strongly curved, causing light rays to focus too soon. Negative spherical aberration occurs when the lens or mirror is too weakly curved, causing light rays to focus too late. Optical designers use aspheric lenses, corrector plates, and achromatic lenses to minimize spherical aberration in various optical systems.
Why does spherical aberration occur in a lens?
Spherical aberration occurs when light rays pass through lenses with spherical surfaces. Incoming light rays strike curved lens surfaces at different angles. Rays passing through larger angles refract more than those at smaller angles. Light focuses at different points on the camera sensor. Spherical aberration reduces image sharpness as a monochromatic imperfection.
Rays focus at different points when passing through a spherical lens. Light rays passing through the outer parts of the lens focus closer to the lens surface. Rays passing through the central part of the lens focus farther from the lens surface. The focal length difference between central and edge light rays defines spherical aberration. A 50 mm focal length lens with a 25 mm diameter experiences significant image quality loss with 1 mm of spherical aberration.
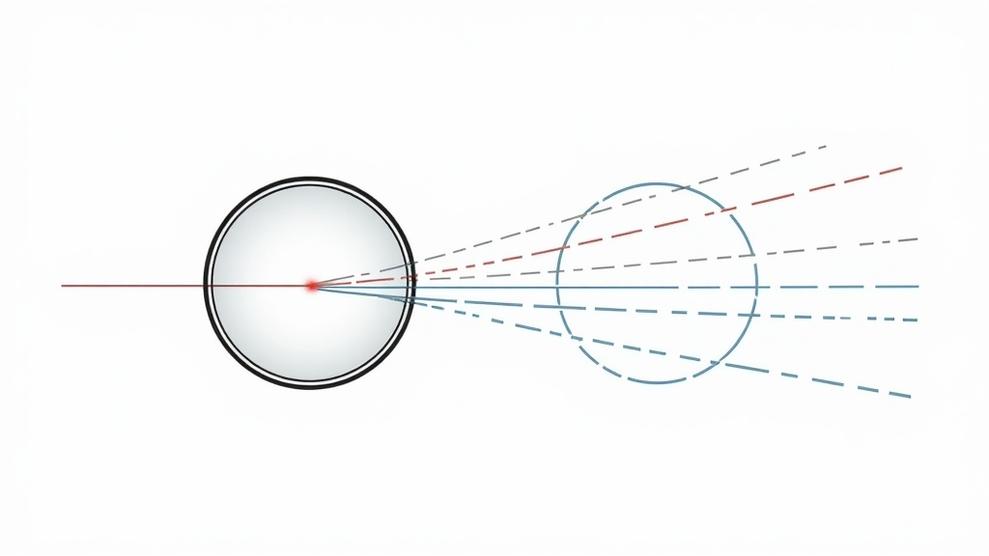
Spherical aberrations result from the lens shape and refractive index differences. The lens material and surrounding medium have different refractive indices. Spherical lens shapes are not ideal for focusing light to a single point. Perfect lenses would converge all light rays at a single focal point. Spherical aberration decreases image sharpness and quality in optical systems.
What is the optical centre of a spherical lens?
Optical centre is the central point of a spherical lens. Light rays passing through this point remain undeviated. Primary axis ray intersects the lens at the optical centre. Lens curvature does not affect light traversing this point. Optical centre sits equidistant from lens edges. Principal axis of the lens crosses at the optical centre.
What is the formula for refraction at a spherical surface?
The formula for refraction at a spherical surface is μv−1u=μ−1r. Refractive index (μ), object distance (u), image distance (v), and radius of curvature (r) are the key components of this equation. The formula establishes the relationship between these variables for light passing through a spherical interface.
Snell’s law forms the basis for deriving the refraction formula. Small angle approximations and angle sum theorems simplify the expression. The center of the sphere serves as the point where the surface is tangent to incident and refracted rays. Focal point is where refracted rays converge or appear to diverge.
Concave surfaces form real and inverted images. Convex surfaces produce virtual and upright images. Refracting power F = (μv – μu) / r measures the surface’s ability to bend light. Positive v values indicate real images, while negative v values represent virtual images.
The formula applies to both convex and concave spherical surfaces using proper sign conventions. Convex surfaces have positive R values, concave surfaces have negative R values. Objects in rarer medium (n1 < n2) produce positive image distances for real images. Objects in denser medium (n1 > n2) result in negative image distances for virtual images.
How to reduce spherical aberration in a thin lens?
Spherical aberration in thin lenses is reduced using multiple techniques. Aperture stops minimize marginal rays entering the lens. Masking blocks marginal rays most affected by spherical aberration. Aspheric lens design incorporates non-spherical shapes. Lens curvature adjustment decreases aberration. Circular annular masks allow only paraxial rays to pass through. Larger curvature radii and lower refractive index materials reduce aberration.
Peripheral ray blocking reduces spherical aberration. Lens combinations effectively block outer light rays that contribute to distortion. Smith (2000) explained how positive lenses combine with negative lenses to reduce spherical aberration. Smaller apertures help reduce spherical aberration by limiting light entry. Hecht (2002) discussed aperture effects on image quality, noting that blocked rays result in sharper images.
Multiple lens elements increase light convergence accuracy. Distributed optical power across several surfaces reduces overall aberrations. Lens doublets cancel out spherical aberrations through the use of glasses with different refractive indices. Kingslake (1983) explained lens combination techniques for pairing positive and negative lenses to cancel spherical aberration. Field flattener lenses decrease spherical aberration by spreading light rays. Smith (2000) described how field flatteners compensate for aberration through controlled ray distribution.
In what conditions does spherical aberration occur?
Spherical aberration occurs in optical systems with spherical-shaped lenses. Light rays entering the lens fail to focus at a single point. Peripheral rays focus differently than central rays. Non-uniform surface curvature causes this aberration. Monochromatic light exacerbates the effect. Imperfections in lenses contribute to spherical aberration. Hartmann and Pearl tests measure this phenomenon. Tracey’s research optimizes lens curvature to minimize aberration.
Optical systems with elements having spherical surfaces are prone to spherical aberration. Lenses operating at maximum aperture exhibit more spherical aberration. Kingslake (1983) notes that spherical aberration is more pronounced when lenses are wide open at their maximum aperture.
Light passes through a refracting surface in optical systems with spherical aberration. Oblique rays enter the lens at an angle during light passage. Hecht (2002) explains that oblique rays are refracted more than central rays by the lens. The lens focuses oblique rays at a different plane than central rays. Malacara (2007) observes that maximum aperture increases the angle of oblique rays.
Spherical aberration telescope experiences significant distortion of images. Schroeder (2000) discusses how telescope lenses or mirrors with spherical surfaces cause light rays to focus at different points. Rutten (2003) explains that spherical aberration is a primary limitation of refracting telescopes. Large apertures in optical systems increase spherical aberration.
Why does spherical spherical aberration occur in a spherical mirror?
Spherical aberration occurs in spherical mirrors due to varying angles of incidence. Light rays entering farther from the optical axis have larger angles of incidence, resulting in shorter focal lengths. Rays closer to the axis have smaller angles, creating longer focal lengths. Multiple focal planes form, causing image distortion. Parabolic mirrors eliminate this issue by focusing all rays to a single point.
Rays do not converge to a single focal point in spherical mirrors. Different focal planes are created for peripheral and central rays. Peripheral rays bend more than paraxial rays. A blurred image results from the variation in focal length across the mirror surface. Image quality decreases as rays converge to multiple points along the optical axis.
Spherical mirrors have an intrinsic defect caused by their geometry. The spherical shape inherently causes light ray deviation from the intended path. Focus point varies depending on where rays strike the mirror surface. Mirror curvature directly affects how light rays reflect and converge. Spherical aberration becomes more pronounced for larger angles of incidence. A typical spherical mirror with a 100 mm focal length demonstrates a 5 mm difference in focal points between central and edge rays.
What is the difference between spherical aberration and chromatic aberration?
Spherical aberration and chromatic aberration differ fundamentally in their causes and effects. Spherical aberration results from the shape of the lens, while chromatic aberration stems from varying light wavelengths. The focus point in spherical aberration changes with aperture size, whereas in chromatic aberration it varies with color. Spherical aberration manifests as a circular blur or halo around the image. Chromatic aberration appears as color fringing or a rainbow effect at high-contrast edges.
Spherical aberration occurs with both monochromatic and polychromatic light sources. Chromatic aberration is significant only with polychromatic light sources. Aperture size primarily affects spherical aberration. Optical material quality mainly influences chromatic aberration. Lens shape directly impacts spherical aberration. Lens design affects both types of aberrations.
Spherical aberrations are classified as undercorrection or overcorrection. Undercorrection places the focus point too far from the lens. Overcorrection positions the focus point too close to the lens. Large apertures exacerbate spherical aberration. Small apertures minimize spherical aberration. High-quality lenses, ED glass, and fluorite minimize chromatic aberration. Convex lenses exhibit more pronounced spherical aberration than concave lenses. Spherical aberration degrades image quality through blurring. Chromatic aberration compromises image quality through color fringing.
Why do telescopes have spherical aberration?
Spherical aberration occurs in telescopes due to spherical lenses and mirrors. Spherical shapes focus light rays at different points based on their angle from the optical axis. Light rays entering at higher angles of incidence focus closer to the mirror or lens. Spherical aberration causes image blurring and distortion, especially for objects near the optical axis.
Spherical aberration affects the entire field of view in telescopes. Both spherical mirrors and curved lenses suffer from this issue. Light strikes curved surfaces at varying angles across the optical element. Perfect focus becomes impossible with a single spherical surface. Wetherell (1980) concluded that achieving a perfect focus is unattainable using a single spherical surface in telescopes.
Primary mirrors in many telescopes are spherical in shape, contributing significantly to aberration. Telescopes using spherical primary mirrors or lenses experience fundamental limitations in image quality. King (1979) described spherical aberration as a significant issue causing image degradation in telescopes with spherical primary optics. Light bends differently at various distances from the mirror or lens centers, resulting in distorted images.
Spherical mirrors suffer more severely than lenses from aberration. Mirror curvature causes light to bend more dramatically at edges than centers. Born & Wolf (1999) explained that light rays striking the edges focus differently than those striking the centers, leading to image blurring and loss of sharpness. The primary mirror or lens shape directly causes spherical aberration in telescopes.